The True Risk of Fumonisins in Swine Production
Karin Naehrer, Product Manager for Biomin, explains the effects of the mycotoxin group, fumonisins, on pigs and how FUMzyme can help piglets overcome the challenge.Fumonisins (FUM) are a group of mycotoxins produced mainly by Fusarium verticillioides and F. proliferatum (Figure 1). The most predominant member among this group is fumonisin B1 (70 to 80 per cent of the total fumonisin content; Krska et al., 2007). As these toxins mainly occur in maize, they represent a serious threat to swine production. The occurrence of fumonisins in corn was once again presented in Biomin's latest worldwide mycotoxin survey 2011 indicating 83 per cent positive occurrences for this mycotoxin with average FUM-contamination of 2,431µg/kg (Rodrigues and Naehrer, 2012).
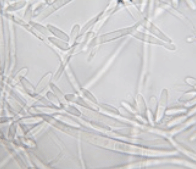
Since the fatal disease outbreak among pigs fed Fusarium verticillioides-contaminated corn, which led to the death of thousands of pigs, numerous experiments have confirmed the link between porcine pulmonary oedema (PPE) and fumonisin exposure (Osweiler et al., 1992; Haschek et al., 2001). Besides severe lung oedemas, liver injuries were also found in the exposed animals. Fumonisin toxicity is based on the structural similarity to the sphingoid bases leading to the inhibition of the biosynthesis of sphingolipids. Based on this, the sphinganine-sphingosine ratio in serum is used as a biomarker for fumonisin exposure, which indicates a major disruption in the sphingolipid metabolism. The main effects in swine are listed in Table 1.
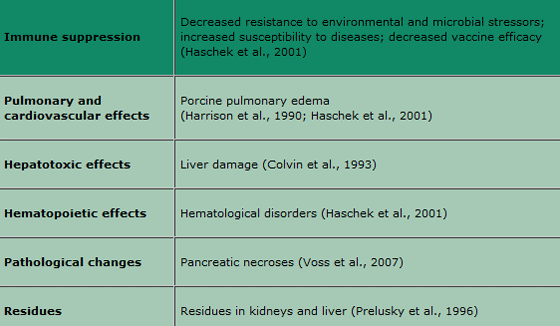
A decline in feed consumption is usually the first sign following fumonisin exposure. In terms of direct effect in weight gain, a study was performed with starter pigs fed diets containing different levels of fumonisin B1. Erratic feeding patterns reflected in the weight and conditions of the stomachs were observed from levels as low as 100ppb. It was postulated that carcass characteristics might also have been affected by this low concentration of toxins (Rotter et al. 1996).
Prior to causing such clinical symptoms, fumonisins often act as immunosuppressive agents. The impacts of these mycotoxins on the immune system of pigs add to the negative economic impact of fumonisins in swine production, and the effects on the specific and non-specific immunity have been reported (Voss, 2007). In the case of vaccination efficacy, feeding weaning piglets with 8mg fumonisin B1 per kg over 28 days led to a significant decrease in antibody titre even after vaccinations against Mycoplasma agalactiae were administered (Taranu et al., 2005).
Fumonisins and Other Interacting Factors
As most fungi are able to produce several mycotoxins simultaneously and feed can be frequently contaminated by different fungi species at the same time, the co-occurrence of mycotoxins is a topic of concern. Additive and more problematic synergistic interactions have been described between fumonisins and other major mycotoxins, which lead to more severe effects in animals. Besides aflatoxins and ochratoxins, combined effects were also investigated with deoxynivalenol, the most frequently detected fusariotoxin (Grenier, 2012).
Data indicate that the ingestion of multi-contaminated diets induces greater histopathological lesions and higher immune suppression (Grenier et al., 2011). It is suggested that deoxynivalenol enhances the intestinal absorption of fumonisins as it is known to be poorly adsorbed. Interactions have been reported not only among different mycotoxins, but also between mycotoxins and other stress factors such as inappropriate farm management, poor feed quality and infectious diseases.
For example, it was found that pigs similarly treated with fumonisins had increased intestinal colonization by pathogenic E. coli (Oswald et al., 2003). In another study, it was suggested that the consumption of fumonisin B1 may predispose piglets to the development of lung inflammation induced by Pasteurella multocida (Halloy et al., 2005). Chronic exposure to fumonisin B1 can decrease the proliferation of undifferentiated porcine epithelial intestinal cells, altering the integrity of intestinal epithelium and consequently facilitating the entry of pathogens into the body (Bouhet and Oswald, 2005).
Fumonisin Counteraction
Preventive measures describe all the steps required to counteract mycotoxins during the growth of the grain as well as during harvesting or storage. Nevertheless, preventive measures can only reduce but not eliminate totally the risk of mycotoxin contamination. Therefore successful detoxification procedures after harvest are essential. As fumonisins are natural toxins, an effective way to counteract them is through biodegradation by natural metabolic pathways, a process called biotransformation. Compared to the adsorption of mycotoxins by clay, microbial biodegradation has the advantage of being highly specific and irreversible.
As for the deactivation of fumonisins, Sphingopyxis MTA 144 is a bacterium capable of the biotransformation of fumonisin B1 into non-toxic metabolite (Hartinger et al., 2011). Heinl and co-workers (2010) provided a basis for the development of an enzymatic detoxification process for fumonisin B1 in food and animal feed. The carboxylesterase FumD (FUMzyme®) is encoded as a part of a gene cluster of Sphingopyxis sp. MTA 144 and enables the bacterial strain to degrade fumonisin B1 (Heinl et al., 2010) into non-toxic metabolite hydrolyzed FB1 (HFB1) (Hartinger and Moll, 2011). Moreover, a recently published study demonstrated that HFB1 does not cause intestinal or hepatic toxicity in the sensitive pig model and only slightly disrupts sphingolipids metabolism. This suggests that conversion to HFB1 could be a good strategy to reduce FB1 exposure (Grenier et al. 2012).
The efficacy of a fumonisin-degrading enzyme, FUMzyme (FDE), in piglets diets contaminated with fumonisin B1 (FB1) was investigated further in in-vivo experiments.
Over a span of 42 days, 30 growing piglets with an initial weight of 11.6kg were kept under controlled conditions. The animals were fed and given water ad libitum. Animals were randomly divided into five treatments and six replicates according to the experimental design. Diets differed only in the inclusion of fumonisin B1 (50ppm) and FDE. FUMzyme (75U or 150U per kg) was mixed in the feed using a bentonite carrier (2.5 or 5kg per tonne of feed).
Parameters measured included zootechnical performance, feed intake, weight gain, feed conversion rate (FCR), relative weight of organs (liver and lungs) and the sphinganine-sphingosine ratio (Sa/So). The parameters and characteristics measured were submitted to variance analysis (ANOVA). Differences between averages were compared by Tukey’s test (P<0.05).
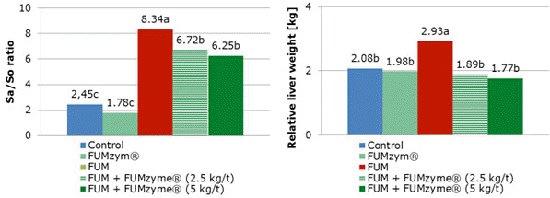
Daily feed intake (kg/animal/day) of animals ingesting fumonisins alone was reduced during the experimental period, from the second week of evaluation. The animals which consumed fumonisins and both FDE-concentrations showed higher daily feed intake compared with FUM-fed animals. The worse results for FCR, relative liver weight and Sa/So ratio were found in animals fed FB1 alone. The inclusion of FUMzyme to the contaminated diet improved these parameters (Figures 2 and 3). The efficiency of FUMzyme against the FB1 challenge in piglets was proven at both inclusion rates (2.5 or 5kg per tonne of feed).
One thing is definite: fumonisins represent a major risk to animal health and performance. Incorporating their counteraction in a mycotoxin risk management approach is therefore of utmost importance.
April 2013